Microbial Food Webs
Importance
Life in the ocean is microbe based. Most
of the biomass and biological activity in the ocean is microscopic,
and microbes dominate earth's carbon, oxygen, sulphur,
and nitrogen cycles. There are 100 million times more bacteria in the
ocean than stars in the known universe, and there are a thousand times
more viruses than bacteria. Yet we know little about the relationships
between microbes and their environment because only one tenth of one
percent of the bacteria have ever been cultured. The traditional
marine food web exists
in a sea of microbes and dissolved carbon-based matter.
The pelagic food web is microbe-centric...The ‘diatom-copepod-fish’ food
web is a relatively minor component.
Barber & Hilting
(2000).
The entire microbial food web, including protozoan
microzooplankton, is typically some five to ten times the mass of all
multicellular marine organisms. The potential metabolic dominance of
microorganisms is even greater than their biomass suggests ... Put in
more understandable terms, a mass of B. natrigens [a bacteria] equal
to 100 humans would have an energy throughput of about a gigawatt, much
the same as a nuclear power station. This metabolic potential under optimal
circumstances would be rarely, if ever, achieved in nature for a number
of reasons, notably the low concentrations of organic nutrients ...
Pomeroy et al (2007).
The microbial food web...has been a major
theme of biological oceanography over the past two decades…
Ducklow (2000).
One approach to understanding the
relationships between micro-organisms and their ocean environment is to examine
their RNA and DNA using, for example, the shotgun
technique whereby DNA is removed from cell samples and forced through
a tiny nozzle to break the DNA into millions of fragments. In 2003, Craig
Venter and colleagues used the technique to analyze the DNA sequences
of micro-organisms in water collected
from the Sargasso Sea near Bermuda and found
1800
genomic species based on sequence relatedness, including 148 previously
unknown bacterial phylotypes. We have identified over 1.2 million previously
unknown genes represented in these samples, including more than 782
new
rhodopsin-like photoreceptors. – Venter et al (2004).
Read more about Venter's ocean work in the Wired Magazine article Craig
Venter's Epic Voyage to Redefine the Origin of the Species
and Carleton University's web pages on marine
microbes. Another approach has been to measure the number of microbes, their productivity,
and the amount of particulate matter in the water to calculate how much
the microbes contribute to various parts of the oceanic food web. This
approach shows that microbes, especially the picoplankton, microbial
plankton with sizes ranging from 0.2 to 2.0 microns, are as important
as the traditional marine food web.
The microbial food web (loop on the left) is an important part of the
marine food web. Picoplankton feed larger zooplankton directly
(path 6) and indirectly (paths 3, 4, and 6), and they contribute to
particulate and dissolved carbon in the ocean. Click on image for a
zoom.
From Barber
(2007).
The Microbial Food Web Is Mostly Unknown
The staggering variety of microbes discovered through shotgun DNA analysis
shows how little we know about the microbial web. Craig Venter, in
a second voyage of discovery from New England to the southwest Pacific,
returned with billions of bases of DNA sequences.
Venter’s team has
found evidence of so many new microbial
species that the researchers want to redraw
the tree of microbial life... [They] identified
more than 400 microbial species new to
science... The vast majority
of the microbes that found themselves snared in Venter’s filters
were genetically unique... Based on their best guess as to the beginning
and end of each gene teased out from the DNA
sequences, Venter Institute computational
biologist Shibu Yooseph and his colleagues
have concluded that the DNA encodes
6.12 million hypothetical proteins. That finding
almost doubles the number of known proteins
in a single stroke... Most of the
predicted proteins are of unknown function,
and a quarter of them have no similarity to any
known proteins... By comparing predicted amino acid
sequences with those of known proteins, they
found a surprising abundance of signaling proteins
thought to be used only by multicellular
organisms. Among the hypothetical proteins
from their marine samples, the researchers
found 28,000 of the so-called eukaryotic protein
kinases, as well as another 19,000 of a
group that are highly similar to these kinases—
triple the number previously known...
From Ocean Study Yields a Tidal
Wave of
Microbial DNA by Bohannon (2007).
Read that quote again. One 10-month expedition that sampled the ocean
at just 41 locations returned with hundreds of new species able to make
millions of proteins that were previously unknown, including tens of
thousands that were thought to occur only in higher, multi-celled organisms.
And this is from only a tiny part of the ocean.
Clearly, the microbial web is almost unknown. A vast new frontier of
life awaits discovery.
With this in mind, here is a brief outline of what we have learned so
far.
Organisms Comprising the Microbial Food Web
The microbial web includes bacteria and archaea (prokaryotes
without a cell nucleus) and very small eukaryotes (organisms
having cells with a nucleus), ranging in size from 0.01 micrometers
for viruses to 20 micrometers for ciliates, living in a dilute broth
of carbon-based matter ranging from individual molecules to tangled polymers
to colloids to clumps of dead matter called marine snow.
Here is an impressionistic view of microbial life in the sunlit waters
of the ocean.-2.gif)
The microbial
loop: impressionist version. A bacteria-eye view of the
ocean's sunlit layer. Seawater is an carbon-based matter continuum, [a
scientific way to say "soup"], a
gel of tangled polymers with embedded strings, sheets, and bundles
of fibrils and particles, including living organisms, as "hotspots." Bacteria
(red) acting on marine snow (black) or algae (green) can control
sedimentation and primary productivity; diverse micro-niches (hotspots)
can support high bacterial diversity.
From Azam
(1998).
- Viruses, including bacteriophages Most
of the biomass in the ocean is made up of viruses–Zimmer
(2006). They attack and kill bacteria, archaea and other micro-organisms,
which releases the dead's cell contents, adding to the carbon-based
material in the water, and they are able to manipulate the evolution
of other microbial life in the ocean in remarkable ways. There
are more than 5,000 different types of viruses in 100 ml of seawater,
and more than 1,000,000 in a kilogram
of sediment (Rowher 2009).
Patrick Forterre, an evolutionary biologist
at the University of Paris-Sud in Orsay, France, believes that viruses
are at the very heart of evolution. Viruses, Forterre argues, bequeathed
DNA to all living things. Trace the ancestry of your genes back far
enough, in other words, and you bump into a virus. – Zimmer
(2006).
Viruses can be considered to be on the threshold
between living and non-living. In a sense, they can cause themselves
to be reproduced, so they could be considered as being "alive". But they can
only do this by using the machinery of a host cell. Without a host
they exist only as inert, almost crystal-like, "non living" particles
in the environment.
They range generally from 17 to 1000 nm in size (a nanometer = 10-9m). Viruses
are generally host specific, that is a given virus will generally affect only
a certain species or group of related species, or even only certain kinds of
cells within a species.
From Background
information on Viruses, Bacteria and Prions at Marine Science
Online Magazine (no longer in production).
...ocean waters contain about ∼ 4 × 1030 viruses.
Because a marine virus contains about 0.2 fg [1
fg = 1 femto-gram = 10-15 gram] of carbon
and is about 100 nm long, this translates into 200 Megatons of carbon
in marine viruses. If the viruses were stretched end to end they would
span ∼ 10
million light years. In context, this is equivalent to the carbon in ∼ 75
million blue whales (∼ 10% carbon, by weight), and is ∼ 100 times the
distance across our own galaxy. – Suttle, C. A. (2005).
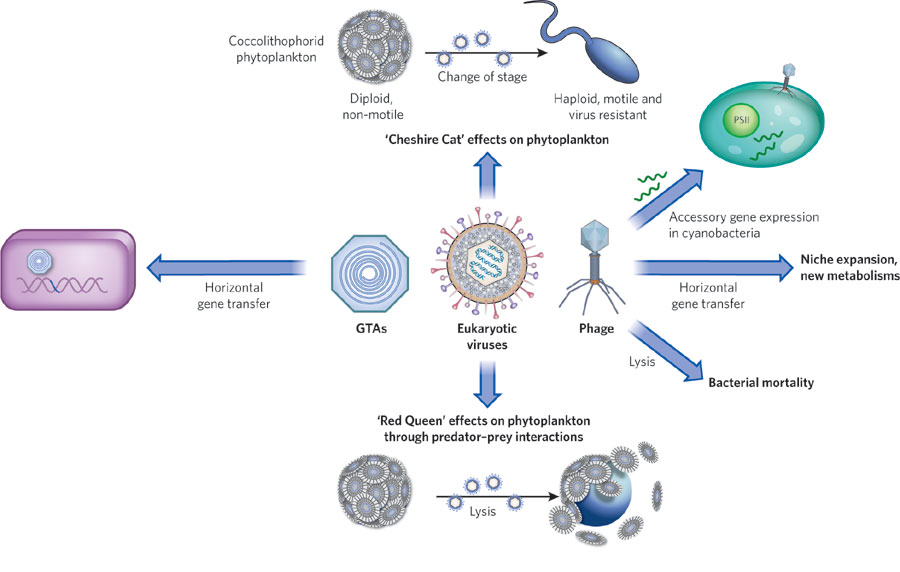
Marine viruses and viral-like entities, including eukaryotic
viruses, phage and generalized transducing agents (GTAs), can have various effects
on host cells. When a phage infects its bacterial host cell, it can either kill
the cell (lysis), or transfer genetic material obtained from a previous host
(horizontal gene transfer) or from its own genome (accessory genes expression).
The transferred genes can allow a cell to expand into different niches (for example,
through the activation of photosynthetic genes, changing the life cycle of important
phytoplankton such as cyanobacteria and coccolithophorids). Similarly, small
viral-like particles known as GTAs can transfer genes between marine organisms.
Two possible scenarios have been proposed for viral effects on cells. In the
'Red Queen' effect, the virus and cell are locked in an evolutionary 'arms race',
such that they continue to evolve mechanisms of resistance to each other until
eventually the virus causes the host cell (illustrated here by a coccolithophorid)
to die. In the 'Cheshire Cat' hypothesis, however, the coccolithophorid simply
moves from its diploid, non-mobile stage to a motile, haploid stage, thereby
evading the virus.
From Rohwer 2009.
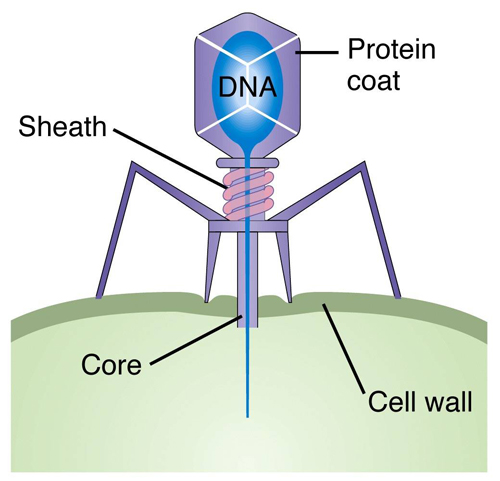
Left: A T4 bacteriophage infecting a bacterium. From An
Introduction to the Bacteriophage T4 Virus at dFORM.
Right: A cartoon of a typical bacteriophage. From
Molecular nanomachines at the
Department of Biochemistry, University of Zurich.
To learn more about how a phage can inject DNA into a cell read The
Physics of Phages (Gelbart and Knobler, 2008)
- Bacteria The ocean is filled with
a countless mob of bacteria with a staggering variety of genes according
to Bohannon (2007). The bacteria are a critical part of the marine
food web, processing more than half of all the flow of carbon-based
matter. Some use sunlight for energy to synthesize hydrocarbons, the
photosynthetic bacteria, some use both sunlight and carbon-based material
for energy, the mixotrophs, and some attack other organisms, the heterotroph
(see the vocabulary section below). The photosynthetic bacteria produced
the oxygen and reduced carbon (carbohydrates) starting billions
of years ago. As carbon was buried in sediments, oxygen accumulated
in the atmosphere (see Role of Ocean
in Climate).
The total mass of bacteria in the ocean exceeds
the combined mass of zooplankton and fishes.– Pomeroy
et al (2007).
It [was] generally thought that pelagic bacteria
passively receive dissolved carbon-based matter leaking from the
grazing food chain and diffused homogeneously. However, recent studies
on the complex nature of the carbon-based matter field and behavioral
strategies of bacteria have suggested that bacteria do not
use only preformed dissolved carbon-based matter; they also attack
all carbon-based matter, even live organisms, thus liberating dissolved
carbon-based matter. Hence, bacterial attack can profoundly modify
the biogeochemical behavior of carbon-based matter in ways not inferred
from measuring cumulative carbon-based matter fluxes into bacteria.
From Azam
(1998).
- Cyanobacteria are the most common type of bacteria in the ocean,
and SAR11 (Pelagibacter clade
[group of closely related organisms] of
the Synechoccus types of bacteria) is the most common organism on
earth, accounting for roughly 25% of all the bacteria in the ocean. Prochlorococcus and Synechoccus species
dominate the microbial ecology of the ocean. They account for 25%
of global photosynthesis. Yet, they were mostly unknown until Sallie
W. Chisholm, a professor at the Massachusetts Institute of Technology,
first discovered
Prochloroccus in 1988.
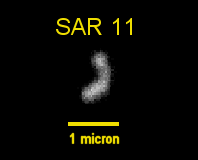
The marine bacterium SAR11, the most common organism
on earth. It was discovered by Stephen Giovannoni of Oregon State University
in 2002.
From Stephen
Giovannoni, Oregon State University, Department of Microbiology.
-
Vibrio bacteria Although bacteria
are very common in the ocean, very few cause disease in people. But
some are deadly.
- The marine bacteria found in most coastal waters, Vibrio
cholerae,
causes cholera.
- Vibrio
vulnificus, which is also found in
coastal waters, including Texas lagoons, causes a flesh-eating
disease (necrotizing
fasciitis) that can kill in a few days without prompt treatment.
If you have a cut that becomes infected after
you were in warm lagoon water, go directly to an emergency
room, especially if the infection spreads. Some
infections can increase from the size of a nickel to the size
of a quarter in a few hours. The
bacteria causes roughly 500 infections and 40 deaths each year
in the USA.
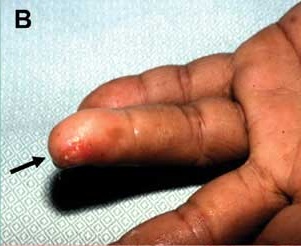
V. vulnificus bacterial infection that
developed one day after a fish bone injury on the fourth finger
of the left hand (arrow) in a 45-year-old patient with uremia.
From Bacteriology
at UW- Madison. More information on Vibrio
vulnificus.
- Archaea They look very much like bacteria,
but they are no more closely related to bacteria than we are to bacteria.
The Archaea dominate some oceanic areas, especially below the sunlit
zones, where "they
make up typically 40% of deep-sea organisms–and
the deep sea is by far the largest habitat on earth" –
Jed Fuhrman, University of Southern California, quoted in Copley (2002).
Overall,
"20% of all the picoplankton cells in
the world ocean appear to be represented by one specific clade, the
pelagic [open-ocean] crenarchaeota". – Karner,
2001.
Archaea are important for earth's nitrogen and methane cycles.
They are important chemotrophes. As far as is known, however, archaea
do not photosynthesize and produce oxygen. But they can use sunlight
as a source of energy.
They also dominate sub-sea sediments:
The deep biosphere is large, and, at least in aggregate, it is 'alive'.
The data almost defy imagination — on the order of a million
cells in every cubic centimeter of sediment buried half a kilometer
below the sea floor, or more than 50% of all microbial cells on Earth.
The overall order of magnitude is the same as the biomass of all surface
plant life. In investigating what kind of cells they are, Lippy
et al. (2008) conclude that, deeper than 1 meter below the sea floor,
it is Archaea, not Bacteria, that contribute the bulk of sedimentary
microbial biomass. If true, Archaea would be the most abundant cell
type in the marine system, rivalling Bacteria in total numbers globally.
From Pearson (2008).
Transmission electron micrograph of Nitrosopumilus
maritimus, (also known as SCM-1), an archae
of the kingdom crenarchaeota. Very similar, open-ocean crenarchaeota
are thought to have an important influence on the nitrogen cycle. Scale
bar represents 0.1 micrometer.
From Nature Article: Isolation
of an autotrophic ammonia-oxidizing marine archaeon by Könneke
et al (2005).
- Chromalveolata This very large group
of single-celled eukaryotes (organisms with a cell nucleus), includes
dinoflagellates, coccolithophores, and diatoms which are important
organisms at the base of the marine-fisheries
food webs, and other organisms, such as
ciliates, described below.
- Dinoflagellates are a large group
of organisms with two, hair-like flagella which they use to swim
through the water. About half of the species are photosynthetic.
Some species occur in dense blooms of more than 109 cells
per liter called harmful
algal blooms, producing potent neurotoxins that accumulate
in marine animals and that kill fish. People become ill or die
when they eat fish or shell fish that have accumulated the toxins.
Some species are the zooxanthellae that live in the cells of corals
and anemones. Others, such as Noctiluca,
produce light when disturbed. Dinoflagellates tend to dominate
in regions of low turbulence and nutrients, such as oceanic areas
in late summer. Sizes range from 30 micrometers for some marine
species up to 2,000 micrometers (2 mm) for Noctiluca.
Leucocryptos marina, a marine dinoflagellate species.
From: Leucocryptos
marina (Braarud) Butcher 1967, Marine
Botany group in the Department of Marine Ecology, Göteborg
University, Sweden.
- Coccolithophores are small organisms
with two smooth flagella and covered by calcareous plates called
coccoliths.They dominate in regions of moderate turbulence and
nutrients such as mid-latitudes in late spring in subpolar regions
and in equatorial regions. Typical size is 5–10 micrometers
in diameter. They are one of the world’s major primary producers,
contributing about 15 per cent of the average oceanic phytoplankton
biomass to the oceans (Berger, 1976). The white cliffs of Dover,
England are made up mostly of coccoliths.
Emiliania huxleyi, a very common marine
coccolithophore.
From Portal
to Protistology.
- Diatoms are described in the chapter on Marine
Fisheries Food Webs.
- Ciliates They
are filter feeders with many hairlike cilia. Some are no larger
than a large bacteria, others can be seen by the naked eye. A paramecium
is a common example of a cilate.
A marine ciliate of the genus strombidium.
From Gulf of
Alaska Plankton Photos.
Some Vocabulary
All organisms need energy, electrons, and carbon (plus associated
nitrogen, phosphorus, and sulphur) to grow and thrive. Organisms can
be classified by how they obtain energy and nutrients. Note -troph means
to nourish. From Greek trophos = "nourishment."
- Autotrophs synthesize their own complex carbon
compounds.
- Phototrophs such as phytoplankton use energy from sunlight plus
carbon dioxide and water to synthesize complex carbon compounds.
- Chemotrophs use energy from inorganic chemical compounds, such
as methane, hydogen sulphide, or reduced iron plus carbon dioxide
or some organic carbon to synthesize complex carbon compounds.
- Mixotrophs are organisms that can use energy
from both sunlight and chemical compounds.
- Heterotrophs are organisms that must eat
other organisms to obtain complex carbon compounds. Some are able to
use sunlight or inorganic compounds for energy, others must use organic
carbon compounds for energy. People are heterotrophs that get complex
carbon compounds and energy from other organisms.
- Bacteriovores eat bacteria.
- Herbivores eat plants.
- Carnivores eat animals.
References
Barber, R. T. and A. K. Hilting (2000). Achievements in biological
oceanography. In: 50 Years of Ocean Discovery.
Ocean Studies Board. Washington DC, National Academy Press: 11–21.
Barber, R. T. (2007). OCEANS: Picoplankton Do Some Heavy Lifting. Science 315
(5813): 777–778.
Bohannon, J. (2007). METAGENOMICS: Ocean Study Yields a Tidal
Wave of Microbial DNA. Science 315 (5818): 1486–1487.
Copley, J. (2002). All at sea. Nature 415: 572–574.
Ducklow, H. (2000). Bacterial production and biomass in the ocean. In:
Microbial Ecology of the Oceans. D. L.
Kirchman, John Wiley: 85–120.
Gelbart, W. M. and C. M. Knobler (2008). The Physics of Phages. Physics
Today 61(1): 42–47.
Karner, M. B., E. F. DeLong, et al. (2001). Archaeal dominance
in the mesopelagic zone of the Pacific Ocean. Nature 409 (6819):
507–510.
Konneke, M., A. E. Bernhard, et al. (2005). Isolation of an autotrophic
ammonia-oxidizing marine archaeon. Nature 437
(7058): 543–546.
Pearson, A. (2008). Biogeochemistry: Who lives in the sea floor? Nature 454
(7207): 952–953.
Pomeroy, L. R., P. J. L. Williams, et al. (2007). The microbial
loop. Oceanography 20 (2): 28–33.
Rohwer, F. and R. V. Thurber (2009). Viruses manipulate the marine
environment. Nature 459 (7244): 207–212.
Sherr, E. and B. Sherr (2000). Marine microbes: An overview. In: Microbial
Ecology of the Oceans. D. L. Kirchman. New York, Wiley-Liss:
13–46.
Shreeve, J. (2004). Craig Venter's epic voyage to redefine the
origin of species. Wired 12 (08): 104–113, 146–151.
Suttle, C. A. (2005). Viruses in the sea. Nature 437(7057):
356–361.
Venter, J. C., K. Remington, et al. (2004). Environmental Genome
Shotgun Sequencing of the Sargasso Sea. Science 304 (5667):
66–74.
Zimmer, C. (2006). Did DNA Come From Viruses? Science 312
(5775): 870–872.
Revised on:
29 May, 2017
|